November 28, 2016
‘Programmable materials’ showing future potential for industry
WEST LAFAYETTE, Ind. – New research has shown that honeycomb “cellular” materials made of a shape-memory polymer might be programmed for specific purposes, from shock-absorbing football helmets to biomedical implants.
“We are introducing a new class of programmable materials whose effective mechanical properties can be modified after fabrication without any additional reprocessing,” said Pablo Zavattieri, an associate professor in Purdue University’s Lyles School of Civil Engineering. “The idea is that you might mass produce the basic material, and it has many potential uses because you can change it later for application A or application B.”
Purdue collaborated with General Motors to develop the materials, which are made of geometric “unit cells” of a shape-memory polymer that can be altered by heating or other methods. The research is detailed in two papers appearing in December in the International Journal of Solids and Structures.
In new findings, the researchers showed they could create programmable cellular materials by introducing deliberate defects to the unit cells. Two types of the honeycomb programmable materials were studied: one having hexagonal cells and the other having cells in a kagome pattern. A YouTube video is available at https://youtu.be/abGAVzueSUc.
“In this case we call defects a good thing because they provide desirable changes in the material cell structure,” said postdoctoral research associate David Restrepo. “This is not intuitive because usually you try to avoid defects. If you have a hexagon, you want the cells to be perfect hexagons. We wanted to look at it another way. We said, if you deformed the hexagon, this could allow you to tune the properties of your material, so these imperfections are actually a good thing.”
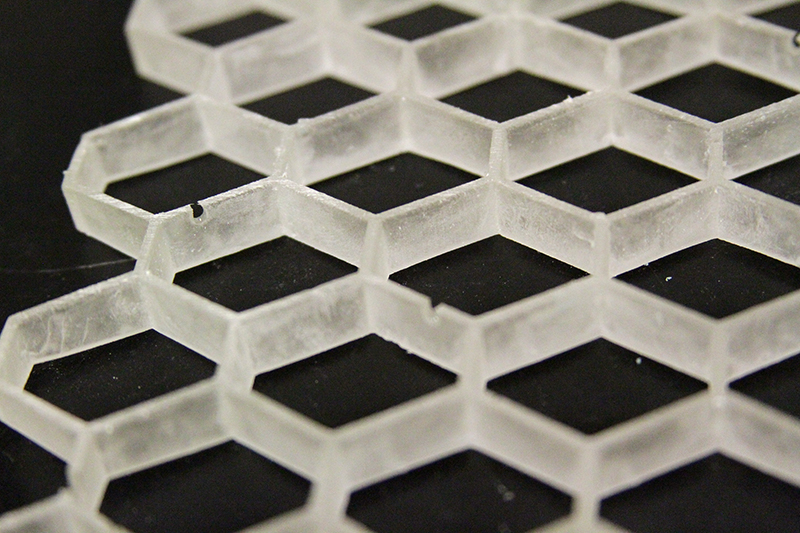
The paper was authored by Restrepo; staff researcher Nilesh D. Mankame from the Smart Materials and Structures Group at the GM Global R&D Center; and Zavattieri.
“There are parallels between the ability to modulate the effective properties of cellular materials by the controlled introduction of carefully chosen imperfections, to the process of doping that lies at the core of low-cost semiconductors, which ushered in the electronics and computing revolution over the last five decades,” Mankame said.
Such an approach might be used for noise-absorbing “acoustic metamaterials” that could be tuned after manufacture to absorb specific frequencies. Other potential long-term innovations include stealthy surfaces that don’t reflect radar waves for military applications, energy-absorbing cushions in football helmets, foams for automotive seating that might be adjusted for specific people based on their weight, and biomedical implants adjusted to match the stiffness of bone and other tissues. The materials might be reprogrammable, as well, meaning they could be altered to suit changing requirements, Zavattieri said.
“Of course, the feasibility of these types of applications may require additional research,” Zavattieri said. “For example, we are not there yet, but say you have a room and you want to shield it from noise. You might put this metamaterial in the walls so that it absorbs certain frequencies. But then say you want to adjust it to cancel out higher frequencies, so you might be able to tune it. It sounds like science fiction, but it’s getting within reach.”
Material properties depend on the shape of the unit cells and the makeup and thickness of the walls separating each cell. Findings showed that compressing the materials by 5 percent results in a 55 percent increase in stiffness, meaning it might be adapted for a range of applications.
“That is pretty impressive because ordinarily you would have to fabricate a new material with at least twice the thickness of the walls to obtain a material with a 50 percent increase on stiffness,” Restrepo said.
Findings also suggest the materials continue to function well if they contain common manufacturing flaws, which suggests that it could be practical for industry.
The researchers also used simulations to study the material, which takes high-power computer clusters to model as many as 10,000 cells, each moving in three directions to simulate bending, stretching and compressing.
“The simulations are very valuable because they allow you to do fewer experiments,” Zavattieri said. “Instead of doing 3,000 experiments, we can do 3,000 simulations, which is much more cost effective.”
The research has been funded by General Motors. Two research papers are available at http://dx.doi.org/10.1016/j.ijsolstr.2016.09.021 and http://dx.doi.org/10.1016/j.ijsolstr.2016.09.022.
Writer: Emil Venere, 765-494-4709, venere@purdue.edu
Sources: Pablo Zavattieri, 765-496-9644, zavattie@purdue.edu
Nilesh D Mankame, nilesh.mankame@gm.com
David Restrepo Arango, drestrep@purdue.edu
Note to Journalists: The two research papers are available at http://dx.doi.org/10.1016/j.ijsolstr.2016.09.021 and http://dx.doi.org/10.1016/j.ijsolstr.2016.09.022. A YouTube video is available at https://youtu.be/abGAVzueSUc and other materials are available on Google drive at http://goo.gl/IlilnL The video was prepared by College of Engineering digital producer Erin Easterling, 765-496-3388, easterling@purdue.edu
ABSTRACT
Programmable materials based on periodic cellular solids. Part I: Experiments
David Restrepo1, Nilesh D. Mankame2, Pablo D. Zavattieri1*
1Lyles School of Civil Engineering, Purdue University
2 Smart Materials and Structures, General Motors Global Research & Development, Warren, MI
*Corresponding author:1+ 765-496-9644, zavattie@purdue.edu (P.D. Zavattieri)
We introduce a new class of programmable materials whose effective mechanical properties can be modified after manufacturing without any additional reprocessing. Programming is achieved by the controlled introduction of a morphological imperfection into the unit cell of a periodic cellular solid, which triggers changes in the effective mechanical properties of these materials. Two programmable material systems are studied in this work: a bending dominated honeycomb with a hexagonal unit cell and a stretching dominated honeycomb with a kagome unit cell. A shape memory polymer is used as the base material and the cellular materials are programmed using the standard shape fixing process for shape memory polymers. We show that significant changes in the effective mechanical properties can be attained with low values of programmed strains. For instance, a programmed imperfection corresponding to a global compressive strain of 5% in the bending dominated system leads to a 55% increase in initial in-plane effective elastic Young’s modulus, an 81% increase in the propagation stress for in-plane compression and a 30% reduction in the out-of-plane effective flexural modulus. The bending dominated system shows a linear change in effective properties with programming up to 10% global programmed strain, whereas the stretching dominated system shows a rapid initial change in effective properties with low values of global programmed strain ( 2% global strain), but the rate of change saturates quickly. Some programmable material systems are amenable to bi-directional modulation (i.e. increase or decrease) of effective properties of the material through suitable programming.