June 2, 2017
Combining MRI and optical microscopy promising for brain research
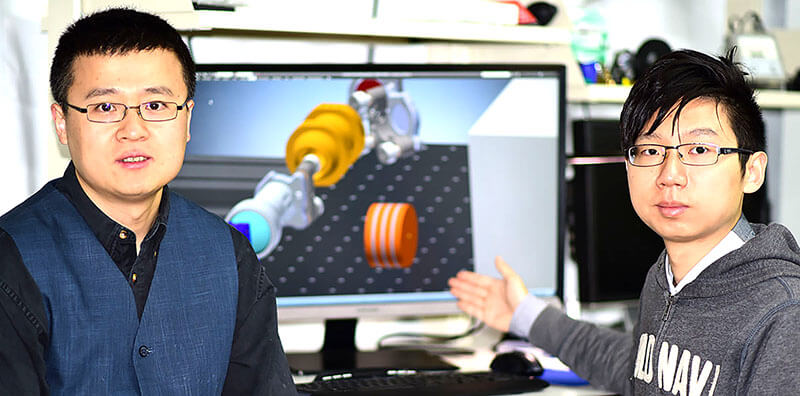
Meng Cui, at left, a Purdue University assistant professor of electrical and computer engineering, and graduate student Yifeng Zhou, display part of a system that allows the simultaneous use of both a functional MRI and “two-photon microscopy” to study the brain. (Purdue University image/Meng Cui)?
WEST LAFAYETTE, Ind. – Functional magnetic resonance imaging reveals changes in blood-oxygen levels in different parts of the brain, but the data show nothing about what is actually happening in and between brain cells, information needed to better understand brain circuitry and function.
“We really have no clear understanding of what cellular processes cause the MRI signal and are left only with hypotheses,” said Meng Cui, an assistant professor in Purdue University’s School of Electrical and Computer Engineering and the Department of Biological Sciences. “We know there is a change in oxygen carried by hemoglobin in the brain, but microscopically nobody knows the details.”
The powerful magnetic fields generated inside MRI machines are detrimental to the delicate electronics needed for sophisticated optical microscopy. Now researchers have solved the problem by designing a system that allows the simultaneous study of brain function with both a functional MRI and a technology called two-photon microscopy. The innovation makes it possible to correlate the MRI signal with microscopic cellular dynamics, which could lead to a better understanding of brain function and the fine neural circuitry, or connectivity.
Researchers from Purdue and the University of Minnesota have demonstrated a proof of concept for the system using a powerful MRI machine.
Findings, appearing Friday (June 2) in the journal Scientific Reports, showed the optical and MRI systems do not interfere with each other, a step toward using the approach for research.
“Understanding human brain function and dysfunction is the key challenge in neuroscience research, and is also essential for the diagnosis and treatment of brain disorders,” Cui said. “However, gaining precise knowledge of neural circuits, the neuronal signaling process and functional connectivity within and among various circuits in the brain relies on innovative and transformative neuroimaging tools.”
Whereas typical MRIs used in clinical medicine have a magnetic field strength of about 3 tesla, the machine at the University of Minnesota’s Center for Magnetic Resonance Research has a field strength of 16.4 tesla.
“This pilot study paved the way for simultaneous ultrahigh-field MRI and high-resolution two-photon microscopy,” Cui said. “This imaging capability will open new opportunities to study and understand the comprehensive relationships between brain structure and connectivity, neuronal activity and dynamics, brain function and cellular energy metabolism in supporting brain function.”
The new system works by relocating the optoelectronics components and laser far from the MRI machine and remotely delivering light to and from the specimen being studied. The research was carried out with a mouse brain, not a living research animal.
The research paper was authored by Cui; Purdue graduate students Yifeng Zhou and Bowen Wei; University of Minnesota researchers Xiao-Hong Zhu, Wei Zhu, Mark A. Sanders, Kamil Ugurbil and Wei Chen.
The research is funded by the National Institutes of Health.
Writer: Emil Venere, 765-494-4709, venere@purdue.edu
Source: Meng Cui, 765-494-5486, mengcui@purdue.edu
Note to Journalists: A copy of the research paper is available online at www.nature.com/articles/s41598-017-02864-0, or from Emil Venere, Purdue News Service, at 765-494-4709, venere@purdue.edu.
ABSTRACT
A proof-of-concept study for developing integrated two-photon microscopic and magnetic resonance imaging modality at ultrahigh field of 16.4 tesla
Meng Cui 1*, Yifeng Zhou1, Bowen Wei1, Xiao-Hong Zhu2, Wei Zhu2, Mark A Sanders3, Kamil Ugurbil2, and Wei Chen2*
1School of Electrical and Computer Engineering, Department of Biological Sciences, Purdue University, West Lafayette, Indiana, 2Center for Magnetic Resonance Research, Department of Radiology, 3Department of Neuroscience, University of Minnesota, Minneapolis, Minnesota
* Corresponding authors: Wei Chen, Ph.D. (Email: wei@cmrr.umn.edu) Center for Magnetic Resonance Research (CMRR) University of Minnesota 2021 6th St. SE Minneapolis, MN 55455 Meng Cui, Ph.D. (Email: mengcui@purdue.edu) 1203 W. State Street Purdue Bindley Bioscience Center Purdue University West Lafayette, IN 47907orrespondence: Dr. Meng Cui, (+1) 765-494-5486 Email: mengcui@purdue.edu
Functional magnetic resonance imaging (fMRI) based on the blood oxygen level dependent (BOLD) contrast has gained a prominent position in neuroscience for imaging neuronal activity and studying effective brain connectivity under working state and functional connectivity at resting state. However, the fundamental questions in regards to fMRI technology: how the BOLD signal inferences the underlying microscopic neuronal activity and physiological changes and what is the ultimate specificity of fMRI for functional mapping of microcircuits, remain unanswered. The capability of simultaneous fMRI measurement and functional microscopic imaging in a live brain thus holds the key to link the microscopic and mesoscopic neural dynamics to the macroscopic brain activity at the central nervous system level. Here we report the first demonstration to integrate high-resolution two-photon fluorescence microscopy (TPM) with a 16.4 tesla MRI system, which proves the feasibility for performing simultaneous high-resolution fMRI and TPM imaging at ultrahigh magnetic field.